There is rapidly growing interest in proton and ion beam therapy for cancer treatment. This is due to the excellent clinical results based on the advantageous depth dose profile of heavy particle beams as well as due to their high radiobiological effectiveness. As has been shown in numerous experiments the radiobiological effectiveness depends both on physical and biological factors. Because of these complex dependencies, biophysical models play a key role in treatment planning systems and radiation protection.
Biological effects of ionizing radiation are mostly dependent on the absorbed dose i.e. energy deposited in a medium per unit mass. It is a fundamental dosimetric quantity well established in numerous dosimetry protocols. However, over the years it becomes more and more clear that such average quantities are not good enough to provide satisfactory predictions. The reason is that fluctuations of the imparted energy play great role in microscopic sensitive sites such as DNA segments. The problem appears when one try to compare outcomes for different types of radiation fields. For such case a new quantity has been introduced, namely the relative biological effectiveness (RBE). It is defined as the ratio of absorbed dose by a reference radiation to the applied radiation for the same biological endpoint (e.g. cell survival).
To understand why different types of radiation have different biological effectiveness, let us use the example presented in figure 1. Multiple damage of the DNA molecule within a short segment of a few nanometres in length is likely to be lethal for the cell. If one imagines a specific absorbed dose administered by photons to a macroscopic volume the interactions will be nearly homogeneously distributed across the target volume. In this case the energy is deposited in a large number of sensitive targets, but each interaction causes rather simple damage, which is not a challenge for repair mechanisms of a cell. For heavy charged particles, on the other hand, the interaction pattern will be very inhomogeneous and the majority of sensitive DNA sites will not be traversed, but some sites will be severely damaged. In result, the probability of cell survival depends not only on the dose, but also on the particle track structure.
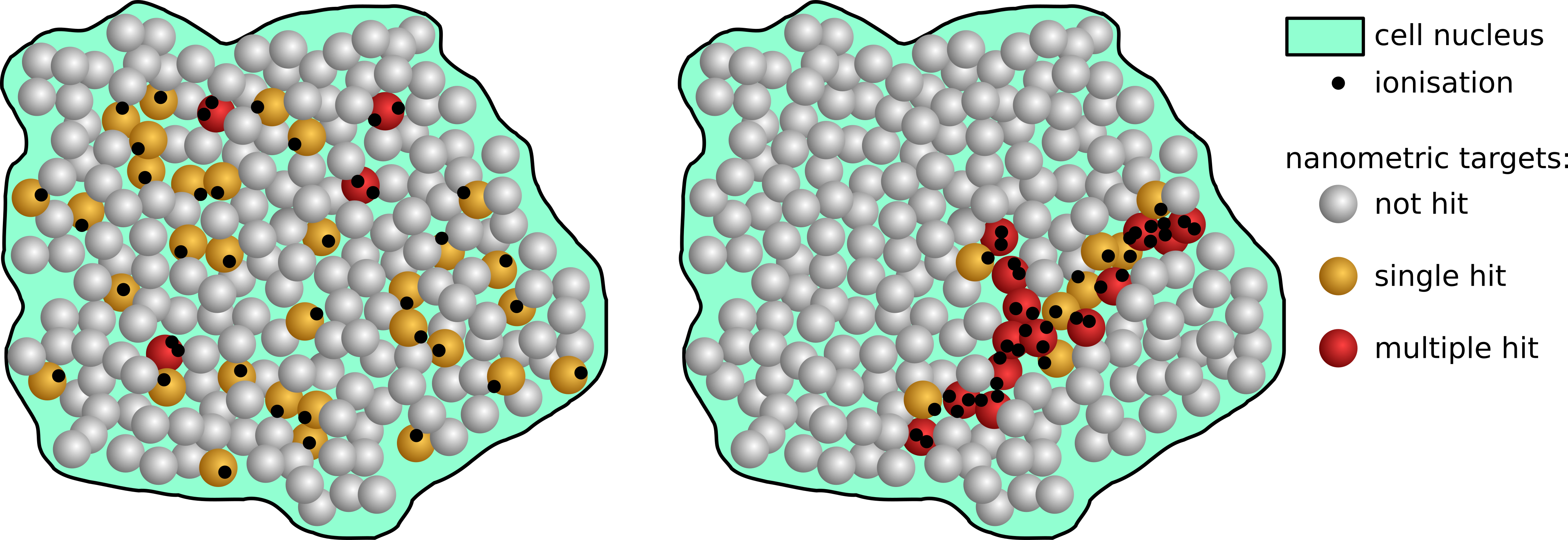
Generally, the RBE of a given radiation is evaluated with time-consuming radiobiological experiments. The growing use of radiotherapy modalities like proton and ion beams raises the need to establish a new dosimetric concept which allows a transparent separation of the physical processes (dependent on modality) from the biological ones (independent of modality) in order to ensure consistency of dose prescription across the different techniques. Both micro- and nanodosimetry have been developed to quantify the physical part of the weighting factors, but each represents a bit different approach. Microdosimetry quantifies fluctuations in the energy transfer to the tissue at the microscopic level, whereas nanodosimetry aims at establishing a new concept of radiation quality that builds on measurable characteristics of the particle track structure (ionisation pattern) at the nanometre scale.
Experimental nanodosimetry is aimed at direct measurement of the ionisations created by a single ionising particle in a nanometric volume equivalent to DNA segments. The target volume can be irradiated directly by a projectile or placed at some distance from the track core where clusters of ions are created only by secondary electrons. In both micro- and nanodosimetry, experiments in gaseous volumes, modelling a condensed target of respective microscopic size, are used in conjunction with Monte Carlo simulations. On the one hand, experimental results can be used as excellent benchmarks for simulations, on the other simulations allow obtaining information that is not available by experimental methods.
The main focus of this project lies on developing experimental methods of particle track structure measurements. Direct measurement in cell environment or in liquid water are not possible. The solution is to prepare equivalent physical system that is experimentally available. Instead of using a condensed phase such as water, the gaseous phase is used in the form of vapors or gases with a similar atomic composition as the living matter (see Figure 2.). In such experiments, the mass of the target is the same, but density is much lower (in order of 0.1-1 µg/cm³ rather than 1 g/cm³).
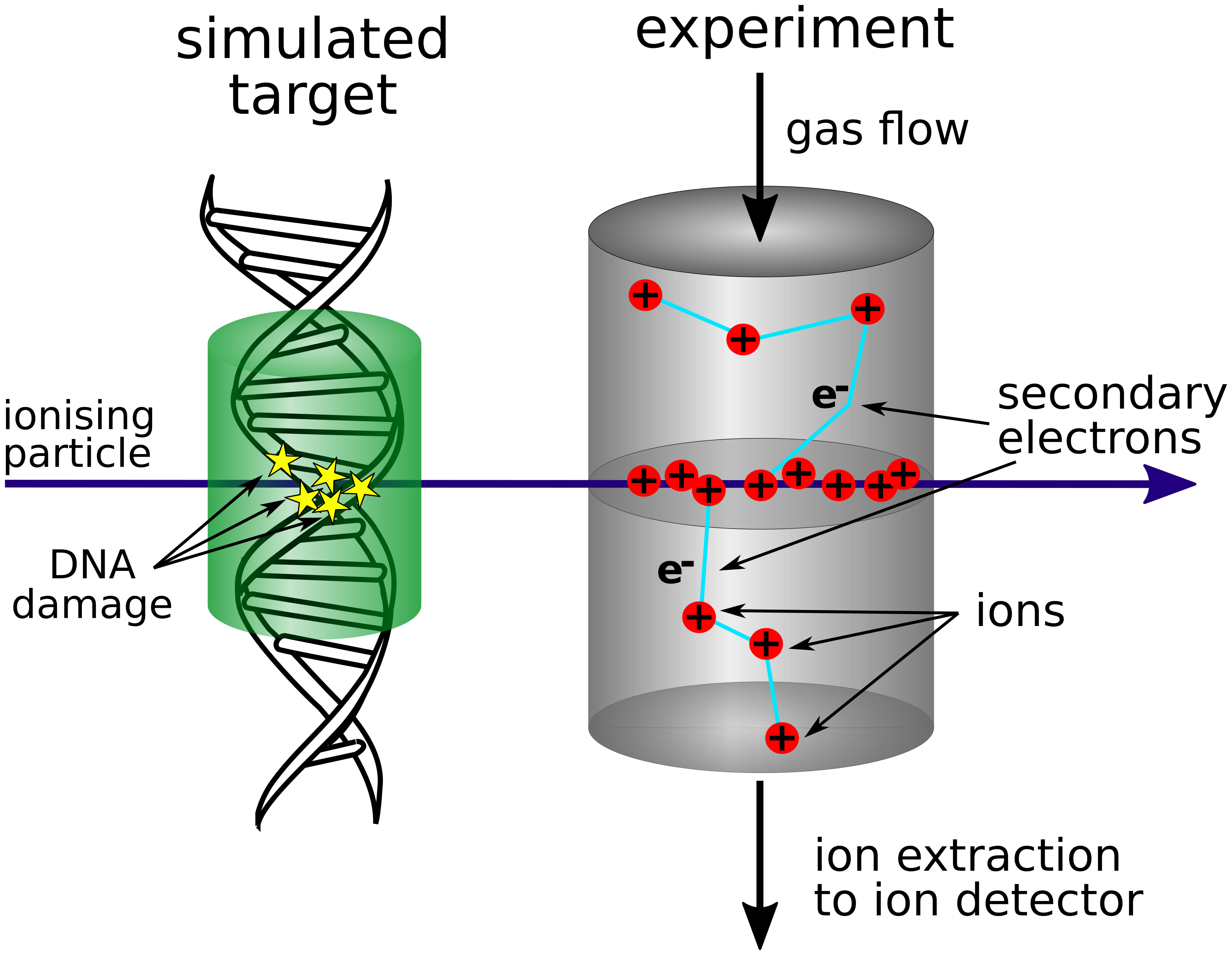
Using Jet Counter nanodosimeter developed at National Centre for Nuclear Research (NCBJ) it is possible to detect single ions produced by ionising particles in gaseous targets of size equivalent to 1-16 nm of liquid water. Currently, a single ion detection efficiency has reached 70-80%. It means that only about 1 in 4 ions created in the target is not detected by the system. The Jet Counter set-up is capable of measuring ionisation clusters (concentrations of ions in the target) for single heavy charged particles. A cluster-size (number of ions) is measured when single particle penetrates the target and hits trigger detector. Number of such events allows for estimation of probability distribution, so called Ionisation Cluster-Size Distribution (ICSD).
Further development is concentrated on extending measuring capabilities to other radiation modalities like photons and low energy electrons. These particles, unlike heavy charged particles, are unable to trigger the system, as each interaction removes them from the beam. Therefore, interaction in the target makes it impossible to hit the trigger detector, and interaction with the trigger means that there was no interaction in the target. In this case the system has to work in non-triggered mode and ICSD for single particle is obtained during data analysis, which require well established detector response function.
The second direction of development is new kind of experiment, where ionisations in two adjacent targets are studied together. One of targets is placed directly in the particle beam while the second one can be irradiated only by secondary electrons produced in the first one. Correlation in size of pairs of clusters can give us very detailed information about production and interaction of secondary electrons, which created ionisation structure around primary particle path.
OUR TEAM
- Marcin Pietrzak, project leader (University of Warsaw, NCBJ)
- Aleksandr Bantsar, mentor (NCBJ)
- Stanisław Pszona (NCBJ)
- Zygmunt Szefliński (HIL)
- Beata Brzozowska (University of Warsaw)
- Mateusz Filipek (University of Warsaw)
- Monika Mietelska (University of Warsaw, NCBJ)